“Many people nowadays think they’re living in a politically polarized era” was the sentence that originally began this post. But the recent resurgence of political violence across all continents, culminating with the attempted assassination of Donald Trump last month, makes that quite literally the understatement of the century. This year alone saw a near-successful attempt on the Prime Minister of Slovakia’s life while dozens of Mexican officials were murdered because of their politics ahead of the country’s June 2nd elections. Former Japanese Prime Minister Shinzo Abe was gunned down by a conspiracy theorist in 2022, and in 2021 both Chad’s President Idriss Deby Itno and Haitian President Jovenel Moïse were killed by those on the opposite side of the political spectrum. These events all beg the question: how long can extremism fester at the poles before we take action?
In times when the human world appears dangerous and unpredictable, I find myself turning to quiet persistency nature and science. Human behavior is notoriously difficult to analyze, especially in the immediate aftermath of a traumatic event when we’d benefit most from self-reflection. Climate science and oceanography aren’t any simpler, of course, but there is a kind of noble objectivity in shifting our attention away from one arena, where information is fickle and limited, to another, where answers abound in their authoritativeness. Part of the reason I have written so little on In Circulation this year is because I’ve waited for moments when the world seemed calmer and the news less dire to improve my chances of being heard (well, read). This strategy, while logical, actually made it harder for me to write, for in moments when my emotions felt most overwhelming I was cutting myself off from the medium in which I could express them.
Since the temperature of global, and specifically American, politics seems unlikely to cool off, it’s time for writers and journalists to rise and meet the moment — and stay at its level for as long as it takes. Trying to talk around the issue of polarization only hinders the overall goal of helping the public understand the full scope of events, whether those events concern divisive politicking or sea ice melting. Dismissing political extremists as too polarized to be taken seriously, or ignoring certain aspects of climate change because of their complexity, will only ensure the root problems take a deeper, less reversible hold. But what I’d like to know is, how long can temperatures be anomalously hot in the poles before permanent damage is done to the entire (eco)system?
Given my degree in oceanography, I will answer that question literally rather than metaphorically. In the past two decades, climatologists have noticed a worrying instability in both Arctic and Antarctic environments: glaciers are melting, sea ice coverage is shrinking and permafrost is thawing, but the hotter temperatures are not evenly distributed. This week on In Circulation, we’ll examine how the Arctic and Southern Oceans are getting the worst of climate change, and how this affects the rest of the globe.
Why are the poles so important?
Scientists have concluded that climate change is happening at an accelerated rate in the North and South Poles. News articles typically cite the metric of twice or three times as fast, but a 2022 study by Rantanen et.al. discovered that warming in the Arctic is actually happening 4x faster than the global average. These scientists accounted for the discrepancy by noting how popular climate models fail to take into account the effect of sea ice on polar climate change. Melting sea ice can actually quicken the already-increased rate of warming; as discussed in a previous newsletter, this causes a positive feedback loop wherein one consequential change (sea ice melt) amplifies the original problem (too much absorption of the sun’s heat makes water temperatures abnormally warm) and makes it worse (way less sea ice forms over time, etc.).
The poles are also home to the ideal conditions for deep water formation. Deep water is denser than surface water because the colder a liquid is, the slower its molecules move and the more nutrients (oxygen, phosphorus, iron, etc.) it can retain. If we recall from a past newsletter, deep water travels across the ocean floor, bringing ‘food’ for plankton from the nutrient-rich, sunlight-deficient poles to the nutrient-deficient, sunlight-rich tropics. Without this cycling of water, the organisms at the base of the food chain would struggle to survive. The cycling of deep water also ensures that there will be room for warm water to continuously flow northward or southward, ensuring a fair distribution of heat across the globe.
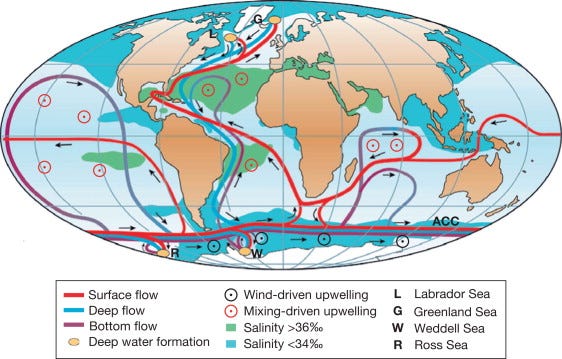
Furthermore, the Arctic and Southern Oceans play host to two climate oscillations (one at each pole). Both the Arctic Oscillation (AO) and Antarctic Oscillation (AAO) are characterized by a natural fluctuation in atmospheric pressure between 40-50ºS and 40-50ºN, roughly speaking. Like the phenomena I discussed in previous editions of my Hot Tub Time Machine series, the AO and AAO are subdivided into positive and negative phases, with the latter bringing mild weather and the former more stormy and chillier winters to the middle latitudes (see graphic below). Although the oscillations have little bearing on the polar regions, the AO and AAO are named after the poles because their mode is entirely dependent on the amount of heat reflected — or absorbed — by the polar environments.
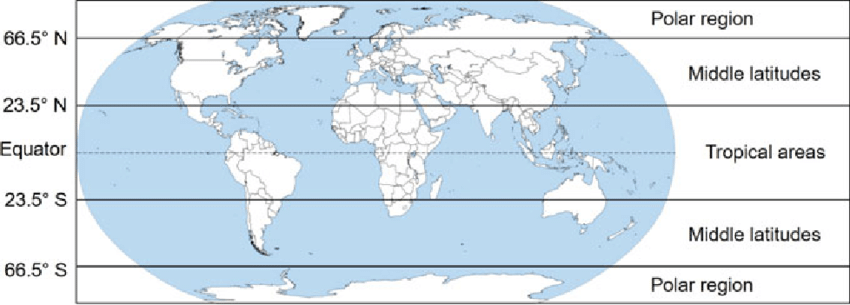
Putting the “polar” in “polarization
Cold air is quite heavy (just like how deep water increases in density the colder it gets), which is why both AO-neg. and AAO-neg. are characterized by extreme high pressure at the poles (high pressure = fewer storms, more consistently cold weather). But if the air is warmer than normal, as it is during the AO/AAO-pos. modes, the pressure over Antarctica and the Arctic decreases dramatically, allowing extra warmth and moisture to linger in those environments. Why does this matter, you may ask? Scientists in 2014 discovered that the AAO has experienced a “progressive shift towards its positive phase.” By examining ice cores dating back to 1000AD(!), they were able to conclude that the AAO has experienced stronger and longer positive phases since the year 1940 because of increased carbon dioxide (CO2) emissions.
This effect has only worsened over time because the Arctic and Southern Oceans trap and store CO2 for longer periods of time than the rest of the planet. In the South Pole, CO2 and other greenhouse gases are stored in Antarctic Bottom Water, which keeps carbon out of the atmosphere for hundreds to thousands of years. Indeed, a 2021 study by Long et. al. found that Antarctic waters absorb a net 530 million metric tons of CO2 — this despite the increased atmosphere and ocean temperatures in the region. By contrast, the Arctic traps carbon in land-based permafrost; since the ground warms at a faster rate than water, the North Pole has been thawing more rapidly than the South. The melting of permafrost renders that environment a net emitter of greenhouse gases, which leads to more melting and thawing of waterways and landscapes and explains why the Arctic Ocean is warming twice as fast as the rest of the oceans.
That climate change happens more quickly and intensely in polar environments is called polar amplification. This concept is nothing new to climatologists, who have realized that the North and South Pole are harbingers of any change in global climate because of Earth’s planetary orbit. Generally speaking, Earth’s rotational speed affects how quickly the ocean and air move on different parts of the earth’s surface: the closer a particle of water or air gets to the poles, the longer it will take to move through that region because the planet spins slower the farther you get from the equator (see graphic below). Normally, this process works to regulate earth’s environments by distributing heat away from the tropics and preventing the polar regions from completely icing over. However, the global air temperatures have increased 0.11°F per decade since the mid-19th century, and average ocean temperatures rose by 0.14°F per decade over roughly the same amount of time. This means the water (in both in the ocean and the clouds) that travels to the Southern and Arctic Oceans nowadays is warmer than it should be; because things move slower at the poles, the heat lingers, thereby increasing the magnitude of environmental change.
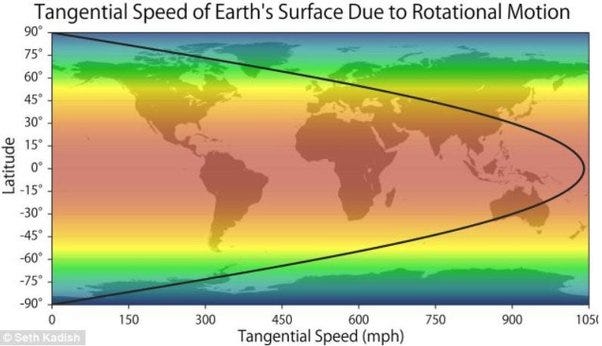
The grand takeaway from all this? Once things start heating up in the poles, not only will it affect the rest of the globe, but it will also take more effort and time to reverse these changes. Most researchers have concluded that polar amplification will have widespread ramifications, although the scientific community is at odds over what exactly they will be. Many predict that the Arctic and Antarctic will become saturated as the climate warms, which would slow down global ocean circulation to devastating effect. Others, such as Yang et. al. 2020, have observed a “poleward shift” of mid-latitude winds, storm tracks, and even large ocean currents because of the increased concentration of heat at the poles (specifically Antarctica). Still others blame localized positive feedback loops, particularly in the Arctic, for quickening the rate of permafrost melt and the rise of air & sea temperatures. In the face of large-scale, high-potential environmental changes, inconclusive science is a bit anxiety-inducing. However, it’s important to discuss uncertainty, since after all one cannot solve a poorly-understood problem.
Welcome to Café Earth
Climate science is complex, but you don’t need to read endless research papers to understand how one small yet consistent temperature change can affect an entire system. Imagine, for example, you work at a café during the summertime. Iced coffees are the most popular item on the menu, and your café is the most popular in town because instead of water ice cubes, you use coffee ice cubes! This is achieved, the owner explains, by pouring the yesterday’s coffee from the pitchers into ice cube molds at the beginning of your shift. These molds are then popped into the freezer and removed after lunch so the afternoon crew has a fresh batch of coffee ice at their disposal. The system works so well because the leftover coffee sits in the refrigerator all night (put there each night after closing time); since it’s already cooled down, it has ample time to freeze before finding its way into someone’s ice-cold drink.
One day you enter the shop at 5:30am and start your opening tasks. After putting several brews on (this is done right away so the coffee will cool off by the time you open at 6:30am), you head to the fridge to make coffee ice, but to your dismay, the pitchers are not in there! You rush over to the counter and discover yesterday’s coffee sitting out, waiting to be served. After taking a moment to curse the closing staff, you quickly pour the room-temperature coffee into the ice cube molds and place them in the freezer. However, when you remove the molds after lunch you find the ice cubes are soft at the edges because even though the coffee was frozen for the same amount of time, it did not freeze completely because its starting temperature was higher than normal. The café’s customers soon begin complaining loudly about slush in their coffees, scaring away passersby, which leads to a loss in revenue and an unhappy boss. Despite your protests, you are unfairly blamed for Ice Cube Gate, as it comes to be known, and instructed to do better in the future.
Tomorrow is a new day, yet it brings the same problems: the leftover coffee sits menacingly on the counter instead of in the refrigerator. This time, however, you’re prepared: you pour the room-temperature coffee into the molds and pop them into the freezer, but instead of taking them out after lunch, you sit around and wait an extra hour to ensure the coffee ice is fully frozen before bringing it out to serve. Because of your malicious compliance, the afternoon rush takes far longer to wind down (not to mention the owner owes you an extra hour of pay!), but customers seem fine with waiting longer for their perfect iced coffee — for now. As long as this delay happens only on occasion, the general flow of business will remain the same, and any money the boss loses will be made up in the near future when everything gets back on schedule… unless, of course, there’s a more permanent change in the (work) climate.
Our planet is a bit like this coffee shop: able to adapt to any slow changes and in need of extra time to adjust to sudden ones. We’d like to think that after one disaster (one Ice Cube Gate) everyone would realize their mistake and work to prevent a similar thing from happening again. But what would happen if your fellow employees get used to doing things improperly (after all, it’s easier on them to let the coffee stay warm)? Or if the refrigerator breaks, eliminating the area of medium-coldness in the kitchen? One change can amplify the next, making the (eco)system increasingly polarized until it either reaches a natural tipping point or until someone steps up and starts reversing the negative changes. Let’s hope, in all cases, that it’s the latter.
Additional sources
US News 21st Century Political Assassinations | National Snow and Ice Data Center | Climate.gov "Polar Opposites" | Natural Resources of Wales dissolved oxygen | NOAA plankton | NASA Penn State deep water | Politico complexities of the Antarctic | UCAR Antarctic Peninsula warming | NASA Arctic Ocean emissions
Abram, N., Mulvaney, R., Vimeux, F. et al. (2014) “Evolution of the Southern Annular Mode during the past millennium.” Nature Clim Change vol. 4, pp. 564–569. https://doi.org/10.1038/nclimate2235.
Matthew C. Long et al. (2021) “Strong Southern Ocean carbon uptake evident in airborne observations.” Science, vol. 374, pp. 1275-1280. https://doi.org/10.1126/science.abi4355
Rantanen, M., Karpechko, A.Y., Lipponen, A. et al. (2022) “The Arctic has warmed nearly four times faster than the globe since 1979.” Commun Earth Environ vol. 3, issue 168. https://doi.org/10.1038/s43247-022-00498-3.
Yang, H., Lohmann, G., Krebs-Kanzow, U., Ionita, M., Shi, X., Sidorenko, D., et al. (2020). “Poleward shift of the major ocean gyres detected in a warming climate.” Geophysical Research Letters, vol. 47, pp. 858-868. https://doi.org/10.1029/2019GL085868.
So interesting and informative!! I also like the iced coffee analogy 🙂